Introduction
Generalized tonic-clonic seizure (GTCS) is one of the most prevalent neurological diseases in Iran, with a prevalence of 16.6 per 1000 people [
1]. Hippocampal and extra-hippocampal pathologies contribute to GTCS [
2, 3]. Vascular endothelial growth factor (VEGF) is a neuroprotective agent, and its administration may promote neuronal survival, neurogenesis, and cerebral angiogenesis [
4, 5]. Its inhibitory effect on the firing rate of the action potentials of hippocampal neurons is mediated by modulation of the voltage-gated sodium channel [
6]. The beneficial effects of VEGF on neurodegenerative and neuropathic conditions have been reported in people with neurological disorders such as trauma, seizures, and ischemia [
7]. In an animal study, a decrease in the VEGF level caused a rapid and sustained change in the brain activity of rodents [
8]. Also, VEGF can protect neuronal damage caused by seizures [
9]. Moreover, VEGF has positive effects on neuroinflammation, brain vascular permeability, neurotransmitter release, synaptic function, reactive oxygen species (ROS)-induced cellular damage, and N-methyl-D-aspartate (NMDA) receptor function [
10, 11]. In a study, recombinant human VEGF (rhVEGF) was used as an anti-cancer agent and showed a stimulatory effect on axonal growth and enhanced Schwann cell proliferation and survival [
9,
12, 13]. Its administration can lead to a decline in glutamate excitotoxicity in the cultured hippocampal neurons [
14]. Administration of rhVEGF may exert an anti-inflammatory action and improve neural survival in neuropathological conditions [
15]. Therefore, rhVEGF seems to be an appropriate alternative drug for the prevention and treatment of seizures. Since rhVEGF can pass the blood-brain barrier (BBB), it is distributed throughout the brain [
7]. Moreover, it affects neuronal activity in the central nervous system [
16, 17]. Therefore, further investigation of its potential mechanism of action can be beneficial.
Several studies have demonstrated an increase in hippocampus nitric oxide (NO) level and BBB permeability in neurodegenerative diseases, including epilepsy [
18-
22]. Reduction in brain antioxidant capacity due to neuronal hyperexcitability plays an essential role in epileptogenesis and influences anti-epileptic drug therapy [
23]. Furthermore, microRNA 142-5p is involved in epileptic behaviors [
24, 25]. However, the manipulation of miR-142–5p attenuates pilocarpine-induced seizures in mice [
26]. On the other hand, administration of rhVEGF reduces BBB permeability following focal cerebral embolic ischemia and accelerates neurological recovery in rats [
27]. Therefore, targeting the above-mentioned mediators in the brain can exert both anticonvulsant and proconvulsant effects.
In this study, we hypothesize that administering rhVEGF can prevent GTCS by modulating several mechanisms. We aim to investigate whether rhVEGF has anticonvulsant effect or a proconvulsant effect. We also evaluate the effects of NO, total antioxidant capacity (TAC), and miR-142-5p expression in the hippocampus and alteration of the BBB permeability as rhVEGF’s possible mechanisms of action, on maximal electroshock (MES)-induced seizure in mice.
Materials and Methods
In this study, rhVEGF was purchased from Zeist Pajoohan Mahbob Company (Tehran, Iran). The cDNA of mice was prepared according to the method reported in a previous study [
28]. The L-NG-nitro-L-arginine methyl ester hydrochloride (L-NAME) was used as a non-specific inhibitor of NO synthase. Template DNA from clinical specimens was prepared using the high pure PCR template preparation kit (Roche Diagnostics, Mannheim, Germany) according to the manufacturer’s instructions.
Twenty-four male Swiss albino mice weighing 25-30 g were used in the experimental work. Animals were kept in the Experimental Research Laboratory of Shahed University. Habituation occurred under laboratory conditions at 25°C in seven days, with 12:12 h light-dark cycles. Moreover, the mice received a standard commercial diet and ad libitum water. Mice put in groups of seven in standard polypropylene cages and were given food and ad libitum water and maintained in a temperature-controlled (25±2°C) room with a 12:12 day/night cycle. The mice were randomly divided into five groups: Control group without receiving MES (for molecular test), group 1 received 0.1 M phosphate-buffered saline (PBS) injection for 4 consecutive days followed by MES (for seizure tests), group 2 received intraperitoneal (i.p.) saline injection for four consecutive days followed by MES, and groups 3 to 5 received i.p. injection of rhVEGF at 50, 100 and 150 µg/kg/day, respectively, for four consecutive days followed by MES. The doses were determined based on a previous study [
29].
An alternating current (0.2 seconds, 50 mA at 60 Hz) generated by an stimulator (Borj Sanat, Iran) was used to induce MES seizures. The current was applied by ear-clip electrodes moistened with normal saline [
30]. The latency for the onset of the first clonus and duration of tonic hind limb extension (HLE) were recorded. Immediately after the MES, the mice were quickly decapitated, and their brains were removed from the skull and placed in an ice-cold normal saline solution. Brain tissue samples were homogenized in PBS (4°C, pH=7.4). Then, samples were centrifuged at 4000×g for 10 min at 4°C, and the supernatant was used for various biochemical assays. The TAC level in the homogenate was measured using the Naxifer™ TAC assay kit [
31] purchased from Navand Salamat Company (Urmia, Iran). The NO level in the homogenate was measured by the Griess method.
Total RNA was extracted from brain tissues (1 cm3) using a miRNA assay kit (AnaCell, Ana Cell Tec., Iran) according to the manufacturer’s protocol. Reverse transcription of miR142-5p and cDNA was performed using miRNA cDNA Synthesis Kit (Roche Diagnostics, Mannheim, Germany) and cDNA synthesis kit, respectively. Expression levels of miR-142-5p and a housekeeping gene control (18S rRNA) detected with a TaqMan miRNA assay kit (AnaCell extraction kit), were measured using a thermocycler (Applied Biosystems; Thermo Fisher Scientific Inc., USA), according to the manufacturer’s protocol. Thermocycling conditions were as follows: 95˚C for 30 sec, followed by 35 cycles of 95˚C for 10 sec and 60˚C for 25 sec. Primers were as follows: miR-142-5p, forward 5’-AACTCCAGC TGGTCCTTAG-3’, reverse 5’-TCTTGAACCCTCATCCTG T-3’; and housekeeping gene forward 5’-GCTTCGGCAGCACATATACTA AAAT-3’, reverse 5’-CGCTTCACGAATTTGCGT-3’ [
32].
A separate group of mice was tested for BBB permeability by using Evans blue dye extravasation method [
33]. Mice were decapitated 4 h after MES-induced seizure according to previously published protocol with some modification, and their brains were removed, weighed, and homogenized in 1.0 mL of trichloroacetic acid (50% in pure water), and centrifuged at 10,000 rpm for 20 min. The absorbance of supernatants was read by a microplate reader (SpectraMax M5, Molecular Devices, USA) at 630 nm. The amount of Evans blue dye was quantified according to external standard curve of Evans blue dye concentration (25–2,000 ng/mL) in 50% TCA/ethanol (1:3), and expressed in μg/g of brain tissue.
Statistical comparisons were made using GraphPad Prism software, version 5. To determine the differences in seizure threshold, latency of seizures, and brain TAC and NO levels, one-way ANOVA followed by Tukey’s post hoc test were used. The data were presented as Mean±SEM.
Results
As shown in
Table 1, all animals exposed to MES had GTCS.
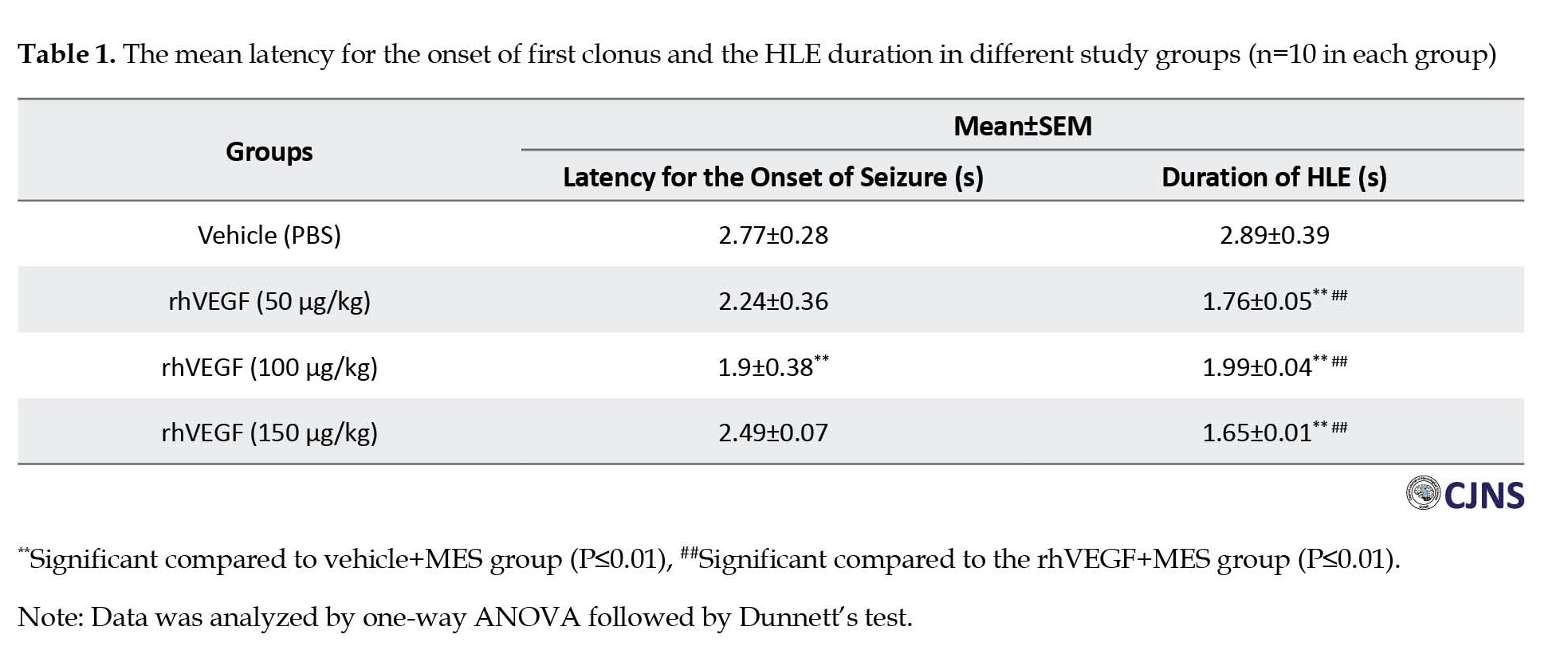
The results showed that the treatment by rhVEGF (50 and 150 µg/kg, i.p.) for four days before MES application had no significant effect on seizure onset. However, treatment with rhVEGF at 100 µg/kg i.p was associated with significantly lower latency for the onset of first clonus. The HLE duration was significantly reduced following treatment with all doses of rhVEGF compared to the MES group (P<0.01).
Compared to the vehicle-treated control group, the NO level significantly increased in the hippocampus of mice after MES-induced seizures (P<0.01). Conversely, treatment with all doses of rhVEGF for four days significantly prevented from MES-induced increase of NO level in the hippocampus (P<0.01,
Figure 1).
As shown in
Figure 2, the TAC of the hippocampus in the MES-induced seizure group was lower (25.26± 0.3 µmol) compared to the control group (30.32±0.27 µmol).
Compared to the MES group, rhVEGF treatment significantly increased the TAC in the brain in a dose-independent manner. The data indicate that treatment with 100 µg/kg dose of rhVEGF increased the TAC more than the 50 and 150 µg/kg doses of rhVEGF.
To investigate the central anti-inflammatory mechanism of rhVEGF in the MES model, the miR-142-5p in the hippocampus, relative to a housekeeping gene expression, was measured by real-time quantitative PCR following MES-induced seizure in mice. The results showed a high expression level of miR-142-5p in the brain of MES-induced seizure group (
Figure 3).
Compared to the MES group, miR-142-5p expression increased significantly in the hippocampus of mice in all rhVEGF-treated groups (P<0.05). These results indicate that rhVEGF enhanced miRNA-142-5p expression in the hippocampus of MES-treated mice.
To investigate the neuroinflammation mechanism of rhVEGF in the MES model, we examined the BBB permeability. The reduction of the leakage showed the improvement of neuroinflammation, indicating the enhancement of BBB leakage following epileptiform activity in the MES group compared to the control group. Treatment with 100 and 150 µg/kg doses of rhVEGF reduced the seizure-induced increase in the BBB permeability (
Figure 4).
Discussion
Our results suggested that rhVEGF may have both proconvulsant and anticonvulsant effects. Decreased myoclonic response was an convulsive response, while reduced HLE duration showed anticonvulsant effect. These results raise questions regarding rhVEGF’s mechanisms of action. A possible explanation for the opposite effects of the same dose of rhVEGF is its dual effect on both presynaptic and postsynaptic mechanisms in the hippocampus [
34]. In support of this hypothesis, a study showed that VEGF dependent mechanisms are likely to be the underlying mechanism for control of balance between glutamate and γ-aminobutyric acid (GABA) in the brain [
35]. Thus, the VEGF in the brain addresses a key challenge in neurobiology of determining how it induces or suppresses effects on neuronal activity to produce proconvulsant or anticonvulsant effects. In addition, molecular studies have shown that VEGF inhibits astrocytic calcium influx, which is a promising therapeutic approach for preventing seizures [
36, 37]. On the other hand, it has been found that cytokines stimulate the production of VEGF, and this compound, in turn, increases seizure potential [
9]. Investigation of the rhVEGF mechanisms identified the modulation of NO level, the increase of TAC and microRNA expression in the hippocampus and the regulation of BBB permeability as the factors that can cause the prevention of seizure development by rhVEGF. In line with this finding, a study suggested that miR-142-5p up-regulation is a natural neuroprotective mechanism against several neurodegenerative disorders [
38]. Therefore, miRNA-142-5p may play a role in rhVEGF’s neuroprotective effect against seizure-induced oxidative stress factors in the brain. As previously reported, VEGF modulates neuronal circuits and suppresses the epileptiform activity in the hippocampus of rats [
35]. It has a regulatory effect on hippocampal neurogenesis and a reverse effect on epileptic seizure in murine [
38]. Furthermore, the VEGF upregulation response following seizures has a protective role in hippocampal neurons [
39]. Thau-Zuchman et al. also showed neuroprotective activity for rhVEGF against traumatic brain injury [
40]. Moreover, the results of a study showed an increase in VEGF and its receptors in the temporal cortex of patients with drug-resistant temporal lobe epilepsy, such that it acted as a proconvulsant [
41]. Additionally, VEGF is related to the increase in the postsynaptic responses mediated by the NMDA receptors in hippocampal neurons [
11]. On the other hand, a study showed that a reduction in the expression of VEGF had an anticonvulsant effect on pentylentetrazole-induced acute seizures in mice [
42]. Blocking the VEGF signaling pathway can reduce the amplitude and width of the action potential in dissociated hippocampal neurons [
43]. Furthermore, VEGF was found to increase the lactate and can increase the excitability of neurons [
44]. These studies suggest that rhVEGF may have a proconvulsant effect.
There are reports regarding the levels of brain NO and oxidative stress markers in epilepsy. Increased NO level and reduced antioxidant capacity can produce anti-epilepsy and may not be merely a consequence of seizures. Electroconvulsive seizures can stimulate the VEGF pathway [
45]. According to Bussolati et al., the VEGF-stimulated NO release is inhibited by the blockade of VEGFR-1 [
46]. Furthermore, it was shown that NO plays an important role in VEGF-induced changes in neuronal activity of the brain in mice [
47]. Similar to another study [
48], we showed the protective effects of rhVEGF on the regulation of BBB permeability. Moreover, several studies showed miRNA-142-5p role in neuronal hyperexcitability in epileptic seizures, oxidative stress, BBB dysfunction, and increased NO level in the brain [
49-
53]. Other subtype of VEGF, such as VEGF-B, has shown potent antioxidant property [
54]. In addition, inhabitation of miRNA-142-5p expression was shown to has an antioxidant effect by reducing the ROS generation in the epileptic rats [
26]. Therefore, the augmenting effect of rhVEGF on miRNA-142-5p expression may be part of its neuroprotective role [
55, 56].
Conclusion
The rhVEGF has both proconvulsant and anticonvulsant effects against MES-induced seizures by modulating NO, TAC, and miRNA-142-5p expression and reducing BBB leakage. Our results provide empirical evidence that rhVEGF has a dose-dependent biphasic effect on MES-induced seizures.
Ethical Considerations
Compliance with ethical guidelines
All experimental procedures were carried out in accordance with the Guide for the Care and Use of Laboratory Animals and approved by the Ethics Committee of Shahed University (Code: IR.SHAHED.REC.1401.058).
Funding
This article was extracted from the master’s thesis of the Morteza Fathi, approved by the Department of Biology, Faculty of Basic Sciences, Shahed University.
Authors contributions
Conceptualization, methodology, investigation, and funding acquisition: All authors; Supervision, resources and writing: Majid Hassanpourezatti.
Conflict of interest
The authors declared no conflict of interest.
Acknowledgements
The authors would like to thank the Deputy for Research of Shahed University for their support in carrying out this study.
References
- Pakdaman H, Harandi AA, Gharagozli K, Alaeddini F, Esfandani A, Mirbehbahani SH, et al. Epilepsy lifetime prevalence in Iran: A large population- based national survey. Sci Rep. 2021; 11(1):9437. [DOI:10.1038/s41598-021-89048-z] [PMID]
- Terrone G, Balosso S, Pauletti A, Ravizza T, Vezzani A. Inflammation and reactive oxygen species as disease modifiers in epilepsy. Neuropharmacology. 2020; 167:107742.[DOI:10.1016/j.neuropharm.2019.107742] [PMID]
- Reschke CR, Henshall DC. microRNA and epilepsy. Adv Exp Med Biol. 2015; 888:41-70. [DOI:10.1007/978-3-319-22671-2_4] [PMID]
- Acosta L, Morcuende S, Silva-Hucha S, Pastor AM, de la Cruz RR. Vascular Endothelial Growth Factor (VEGF) prevents the downregulation of the cholinergic phenotype in axotomized motoneurons of the adult rat. Front Mol Neurosci. 2018; 11:241. [DOI:10.3389/fnmol.2018.00241] [PMID]
- Sun Y, Jin K, Xie L, Childs J, Mao XO, Logvinova A, et al. VEGF-induced neuroprotection, neurogenesis, and angiogenesis after focal cerebral ischemia. J Clin Invest. 2003; 111(12):1843-51. [DOI:10.1172/JCI17977] [PMID]
- Sun GC, Ma YY. Vascular endothelial growth factor modulates voltage-gated Na(+) channel properties and depresses action potential firing in cultured rat hippocampal neurons. Biol Pharm Bull. 2013; 36(4):548-55. [DOI:10.1248/bpb.b12-00841] [PMID]
- Rosenstein JM, Krum JM, Ruhrberg C. VEGF in the nervous system. Organogenesis. 2010; 6(2):107-14. [DOI:10.4161/org.6.2.11687] [PMID]
- Deyama S, Duman RS. Neurotrophic mechanisms underlying the rapid and sustained antidepressant actions of ketamine. Pharmacol Biochem Behav. 2020; 188:172837. [DOI:10.1016/j.pbb.2019.172837] [PMID]
- Croll SD, Goodman JH, Scharfman HE. Vascular endothelial growth factor (VEGF) in seizures: A double-edged sword. Adv Exp Med Biol. 2004; 548:57-68. [DOI:10.1007/978-1-4757-6376-8_4] [PMID]
- Proescholdt MA, Heiss JD, Walbridge S, Mühlhauser J, Capogrossi MC, Oldfield EH, et al. Vascular endothelial growth factor (VEGF) modulates vascular permeability and inflammation in rat brain. J Neuropathol Exp Neurol. 1999; 58(6):613-27. [DOI:10.1097/00005072-199906000-00006] [PMID]
- De Rossi P, Harde E, Dupuis JP, Martin L, Chounlamountri N, Bardin M, et al. A critical role for VEGF and VEGFR2 in NMDA receptor synaptic function and fear-related behavior. Mol Psychiatry. 2016; 21(12):1768-1780. [DOI:10.1038/mp.2015.195] [PMID]
- Peiris-Pagès M. The role of VEGF 165b in pathophysiology. Cell Adh Migr. 2012; 6(6):561-8. [DOI:10.4161/cam.22439] [PMID]
- Sondell M, Lundborg G, Kanje M. Vascular endothelial growth factor has neurotrophic activity and stimulates axonal outgrowth, enhancing cell survival and Schwann cell proliferation in the peripheral nervous system. J Neurosci. 1999; 19(14):5731-40. [DOI:10.1523/JNEUROSCI.19-14-05731.1999] [PMID]
- Matsuzaki H, Tamatani M, Yamaguchi A, Namikawa K, Kiyama H, Vitek MP, et al. Vascular endothelial growth factor rescues hippocampal neurons from glutamate-induced toxicity: Signal transduction cascades. FASEB J. 2001; 15(7):1218-20. [PMID]
- Li J, Chen S, Zhao Z, Luo Y, Hou Y, Li H, et al. Effect of VEGF on inflammatory regulation, neural survival, and functional improvement in rats following a complete spinal cord transection. Front Cell Neurosci. 2017; 11:381. [DOI:10.3389/fncel.2017.00381] [PMID]
- Fabel K, Fabel K, Tam B, Kaufer D, Baiker A, Simmons N, et al. VEGF is necessary for exercise-induced adult hippocampal neurogenesis. Eur J Neurosci. 2003; 18(10):2803-12. [DOI:10.1111/j.1460-9568.2003.03041.x] [PMID]
- Cotman CW, Berchtold NC, Christie LA. Exercise builds brain health: Key roles of growth factor cascades and inflammation Trends Neurosci. 2007;30(9):464-72. [DOI:10.1016/j.tins.2007.06.011] [PMID]
- Mohseni G, Ostadhadi S, Akbarian R, Chamanara M, Norouzi-Javidan A, Dehpour AR. Anticonvulsant effect of dextrometrophan on pentylenetetrazole-induced seizures in mice: Involvement of nitric oxide and N-methyl-d-aspartate receptors. Epilepsy Behav. 2016; 65:49-55. [DOI:10.1016/j.yebeh.2016.08.001] [PMID]
- Chaudhuri AD, Yelamanchili SV, Fox HS. MicroRNA-142 reduces monoamine oxidase A expression and activity in neuronal cells by downregulating SIRT1. PLoS One. 2013; 8(11):e79579. [DOI:10.1371/journal.pone.0079579] [PMID]
- Baloun J, Bencurova P, Totkova T, Kubova H, Hermanova M, Hendrych M, et al. Epilepsy miRNA profile depends on the age of onset in humans and rats. Front Neurosci. 2020; 14:924. [DOI:10.3389/fnins.2020.00924] [PMID]
- Venø MT, Reschke CR, Morris G, Connolly NMC, Su J, Yan Y, et al. A systems approach delivers a functional microRNA catalog and expanded targets for seizure suppression in temporal lobe epilepsy. Proc Natl Acad Sci U S A. 2020; 117(27):15977-88. [DOI:10.1073/pnas.1919313117] [PMID]
- Coulter DA, Steinhäuser C. Role of astrocytes in epilepsy. Cold Spring Harb Perspect Med. 2015; 5(3):a022434.[DOI:10.1101/cshperspect.a022434] [PMID]
- Martinc B, Grabnar I, Vovk T. The role of reactive species in epileptogenesis and influence of antiepileptic drug therapy on oxidative stress. Curr Neuropharmacol. 2012; 10(4):328-43. [DOI:10.2174/157015912804143504] [PMID]
- Xiao D, Lv J, Zheng Z, Liu Y, Zhang Y, Luo C, et al. Mechanisms of microRNA142 in mitochondrial autophagy and hippocampal damage in a rat model of epilepsy. Int J Mol Med. 2021; 47(6):98. [DOI:10.3892/ijmm.2021.4931] [PMID]
- Chen J, Jiang C, Du J, Xie CL. MiR-142-5p protects against 6-OHDA-induced SH-SY5Y cell injury by downregulating BECN1 and autophagy. Dose Response. 2020; 18(1):1559325820907016. [DOI:10.1177/1559325820907016] [PMID]
- Zhang H, Lian Y, Xie N, Cheng X, Chen C, Xu H, et al. Antagomirs targeting miR-142-5p attenuate pilocarpine-induced status epilepticus in mice. Exp Cell Res. 2020; 393(2):112089. [PMID]
- Zhang ZG, Zhang L, Jiang Q, Zhang R, Davies K, Powers C, et al. VEGF enhances angiogenesis and promotes blood-brain barrier leakage in the ischemic brain. J Clin Invest. 2000; 106(7):829-38. [DOI:10.1172/JCI9369] [PMID]
- Tang FL, Zhao L, Zhao Y, Sun D, Zhu XJ, Mei L, et al. Coupling of terminal differentiation deficit with neurodegenerative pathology in Vps35-deficient pyramidal neurons. Cell Death Differ. 2020; 27(7):2099-116. [DOI:10.1038/s41418-019-0487-2] [PMID]
- Rennel ES, Hamdollah-Zadeh MA, Wheatley ER, Magnussen A, Schüler Y, Kelly SP, et al. Recombinant human VEGF165b protein is an effective anti-cancer agent in mice. Eur J Cancer. 2008; 44(13):1883-94. [DOI:10.1016/j.ejca.2008.05.027] [PMID]
- Hu M, Liu Y, He L, Yuan X, Peng W, Wu C. Antiepileptic effects of protein-rich extract from bombyx batryticatus on mice and its protective effects against H2O2-induced oxidative damage in PC12 cells via regulating PI3K/Akt signaling pathways. Oxid Med Cell Longev. 2019; 2019:7897584. [DOI:10.1155/2019/7897584] [PMID]
- Hassan AA, Bel Hadj Salah K, Fahmy EM, Mansour DA, Mohamed SAM, Abdallah AA, et al. Olive leaf extract attenuates chlorpyrifos-induced neuro- and reproductive toxicity in male albino rats. Life (Basel). 2022; 12(10):1500. [DOI:10.3390/life12101500] [PMID]
- Xu W, Wang W. MicroRNA1425p modulates breast cancer cell proliferation and apoptosis by targeting phosphatase and tensin homolog. Mol Med Rep. 2018; 17(6):7529-7536. [DOI:10.3892/mmr.2018.8812]
- Cheng C, Wang X, Jiang Y, Li Y, Liao Z, Li W, et al. Recombinant Annexin A2 administration improves neurological outcomes after traumatic brain injury in mice. Front Pharmacol. 2021; 12:708469. [DOI:10.3389/fphar.2021.708469] [PMID]
- Yang J, Yang C, Liu C, Zhang T, Yang Z. Paradoxical effects of VEGF on synaptic activity partially involved in notch1 signaling in the mouse hippocampus. Hippocampus. 2016; 26(5):589-600. [DOI:10.1002/hipo.22544] [PMID]
- McCloskey DP, Croll SD, Scharfman HE. Depression of synaptic transmission by vascular endothelial growth factor in adult rat hippocampus and evidence for increased efficacy after chronic seizures. J Neurosci. 2005; 25(39):8889-97. [DOI:10.1523/JNEUROSCI.2577-05.2005] [PMID]
- Kou ZW, Mo JL, Wu KW, Qiu MH, Huang YL, Tao F, et al. Vascular endothelial growth factor increases the function of calcium-impermeable AMPA receptor GluA2 subunit in astrocytes via activation of protein kinase C signaling pathway. Glia. 2019; 67(7):1344-58. [DOI:10.1002/glia.23609] [PMID]
- Newton J, Akinfiresoye LR, N’Gouemo P. Inhibition of the sodium calcium exchanger suppresses alcohol withdrawal-induced seizure susceptibility. Brain Sci. 2021; 11(2):279. [DOI:10.3390/brainsci11020279] [PMID]
- Han W, Song X, He R, Li T, Cheng L, Xie L, et al. VEGF regulates hippocampal neurogenesis and reverses cognitive deficits in immature rats after status epilepticus through the VEGF R2 signaling pathway. Epilepsy Behav. 2017; 68:159-67. [DOI:10.1016/j.yebeh.2016.12.007] [PMID]
- Nicoletti JN, Shah SK, McCloskey DP, Goodman JH, Elkady A, Atassi H, et al. Vascular endothelial growth factor is up-regulated after status epilepticus and protects against seizure-induced neuronal loss in hippocampus. Neuroscience. 2008; 151(1):232-41. [DOI:10.1016/j.neuroscience.2007.09.083] [PMID]
- Thau-Zuchman O, Shohami E, Alexandrovich AG, Leker RR. Vascular endothelial growth factor increases neurogenesis after traumatic brain injury. J Cereb Blood Flow Metab. 2010; 30(5):1008-16. [DOI:10.1038/jcbfm.2009.271] [PMID]
- Castañeda-Cabral JL, Beas-Zárate C, Rocha-Arrieta LL, Orozco-Suárez SA, Alonso-Vanegas M, Guevara-Guzmán R, et al. Increased protein expression of VEGF-A, VEGF-B, VEGF-C and their receptors in the temporal neocortex of pharmacoresistant temporal lobe epilepsy patients. J Neuroimmunol. 2019; 328:68-72. [DOI:10.1016/j.jneuroim.2018.12.007] [PMID]
- Kazmi Z, Zeeshan S, Khan A, Malik S, Shehzad A, Seo EK, et al. Anti-epileptic activity of daidzin in PTZ-induced mice model by targeting oxidative stress and BDNF/VEGF signaling. Neurotoxicology. 2020; 79:150-63. [DOI:10.1016/j.neuro.2020.05.005] [PMID]
- Latzer P, Shchyglo O, Hartl T, Matschke V, Schlegel U, Manahan-Vaughan D, et al. Blocking VEGF by bevacizumab compromises electrophysiological and morphological properties of hippocampal neurons. Front Cell Neurosci. 2019; 13:113. [DOI:10.3389/fncel.2019.00113] [PMID]
- Zhou J, Liu T, Guo H, Cui H, Li P, Feng D, et al. Lactate potentiates angiogenesis and neurogenesis in experimental intracerebral hemorrhage. Exp Mol Med. 2018; 50(7):1-12. [DOI:10.1038/s12276-018-0113-2] [PMID]
- Elfving B, Wegener G. Electroconvulsive seizures stimulate the vegf pathway via mTORC1. Synapse. 2012; 66(4):340-5. [DOI:10.1002/syn.21518] [PMID]
- Bussolati B, Dunk C, Grohman M, Kontos CD, Mason J, Ahmed A. Vascular endothelial growth factor receptor-1 modulates vascular endothelial growth factor-mediated angiogenesis via nitric oxide. Am J Pathol. 2001; 159(3):993-1008. [DOI:10.1016/S0002-9440(10)61775-0] [PMID]
- Licht T, Goshen I, Avital A, Kreisel T, Zubedat S, Eavri R, et al. Reversible modulations of neuronal plasticity by VEGF. Proc Natl Acad Sci U S A. 2011; 108(12):5081-6. [DOI:10.1073/pnas.1007640108] [PMID]
- Jiang S, Xia R, Jiang Y, Wang L, Gao F. Vascular endothelial growth factors enhance the permeability of the mouse blood-brain barrier. Plos One. 2014; 9(2):e86407. [DOI:10.1371/journal.pone.0086407] [PMID]
- Marchi N, Granata T, Ghosh C, Janigro D. Blood-brain barrier dysfunction and epilepsy: Pathophysiologic role and therapeutic approaches. Epilepsia. 2012; 53(11):1877-86. [DOI:10.1111/j.1528-1167.2012.03637.x] [PMID]
- De Benedittis S, Fortunato F, Cava C, Gallivanone F, Iaccino E, Caligiuri ME, et al. Circulating microRNA: The potential novel diagnostic biomarkers to predict drug resistance in temporal lobe epilepsy, a pilot study. Int J Mol Sci. 2021; 22(2):702. [DOI:10.3390/ijms22020702] [PMID]
- Sharma S, Puttachary S, Thippeswamy T. Glial source of nitric oxide in epileptogenesis: A target for disease modification in epilepsy. J Neurosci Res. 2019; 97(11):1363-77. [DOI:10.1002/jnr.24205] [PMID]
- Li S, Lei Z, Sun T. The role of microRNAs in neurodegenerative diseases: A review. Cell Biol Toxicol. 2023; 39(1):53-83. [DOI:10.1007/s10565-022-09761-x] [PMID]
- Aguiar CC, Almeida AB, Araújo PV, de Abreu RN, Chaves EM, do Vale OC, et al. Oxidative stress and epilepsy: Literature review. Oxid Med Cell Longev. 2012; 2012:795259. [DOI:10.1155/2012/795259] [PMID]
- Arjunan P, Lin X, Tang Z, Du Y, Kumar A, Liu L, et al. VEGF-B is a potent antioxidant. Proc Natl Acad Sci U S A. 2018; 115(41):10351-6. [DOI:10.1073/pnas.1801379115] [PMID]
- Kweider N, Fragoulis A, Rosen C, Pecks U, Rath W, Pufe T, et al. Interplay between vascular endothelial growth factor (VEGF) and nuclear factor erythroid 2-related factor-2 (Nrf2): Implications for preeclampsia. J Biol Chem. 2011; 286(50):42863-72. [DOI:10.1074/jbc.M111.286880] [PMID]
- Aday S, Zoldan J, Besnier M, Carreto L, Saif J, Fernandes R, et al. Synthetic microparticles conjugated with VEGF165 improve the survival of endothelial progenitor cells via microRNA-17 inhibition. Nat Commun. 2017; 8(1):747. [DOI:10.1038/s41467-017-00746-7] [PMID]